Small modular reactors (SMRs) are gaining increased attention as a major opportunity in clean power production. They are a welcome tool in the necessary transition from an energy system dominated by hydrocarbon combustion to one that produces more power for more people with dramatically reduced greenhouse gas emissions.
As a partner in Nucleation Capital, a venture capital fund that focuses on the commercial opportunities that are anticipated by advanced nuclear technologies and power systems, I thought it would be useful to discuss reasons why so many are eager for the arrival of the growing slate of smaller nuclear reactors that are under development. For important reasons there are few mentions of individual companies.
Like traditional large nuclear reactors, SMRs generate power from fission. They don’t produce any air pollution components like NOX, SOX, mercury or fly ash. Unlike large reactors, which were built as large infrastructure projects, the expectation is that SMRs can be constructed more quickly from prefabricated components so that they can be rolled out at an increasing rate once they have completed a necessary product demonstration phase.
Some energy observers see SMRs as the appropriate evolution of nuclear for use with a more distributed grid. Others believe the sector has been widely “hyped” in online energy forums but won’t be available in the time frame needed to address climate change. So how should those for whom the term SMR is just now entering their lexicon think about this technology?
Why does fission excite anyone?
Uranium and thorium are two of nature’s most incredible clean energy storage assets. If completely fissioned, a handful of nuclear fuel weighing a kilogram contains more stored energy than 50 large tanker trucks filled with petroleum.
At the current diesel fuel price of $5.60 per gallon, 50 trucks can carry more than $3,000,000 worth of fuel. In contrast, nuclear power plant owners pay approximately $1,700 per kilogram of fuel in the form of finished assemblies.
The tiny waste production per unit energy released is an inherent aspect of concentrated fission reactions. Unlike combustion, all ingredients needed for fission are contained inside fission fuels. (Combustion needs an external source of oxygen in greater masses than the fuel itself.) The mass of fission wastes is slightly less than the mass of fission fuel; the mass of combustion wastes are about 2.5 times the mass of input fuel.
No fission product wastes need to be routinely removed to allow the reaction to continue operating for its design fuel cycle. None need to be discharged to the environment. Fission reactors are clean enough, safe enough and independent enough to operate inside sealed submarines carrying crews of several dozen people. Those submarines have gone to every part of every ocean on the planet.
Fission even works in the vacuum of deep space.
Those physical and economic facts almost beg power plant designers to think about building a wide variety of machines in order to use that amazing source of energy in as many parts of the diverse global energy markets as possible. Power systems using combustion fuels range in size from model trains to multi GWe power stations. Fission-based power systems need sufficient size to support a chain reaction, and to provide adequate shielding, but that still leaves a wide spectrum of potential applications and sizes.
Today, advanced nuclear innovators are designing reactors that will meet the needs of a much broader range of energy users than traditional nuclear could previously address.
Why did fission reactor unit size get so large?
The earliest reactors were small; the core of EBR-I (Experimental Breeder Reactor I) was roughly the size of cylinder that could snuggly contain an American football. It produced a 1.5 MW of heat and 200 kW of electricity. That was enough to power the building that housed it.
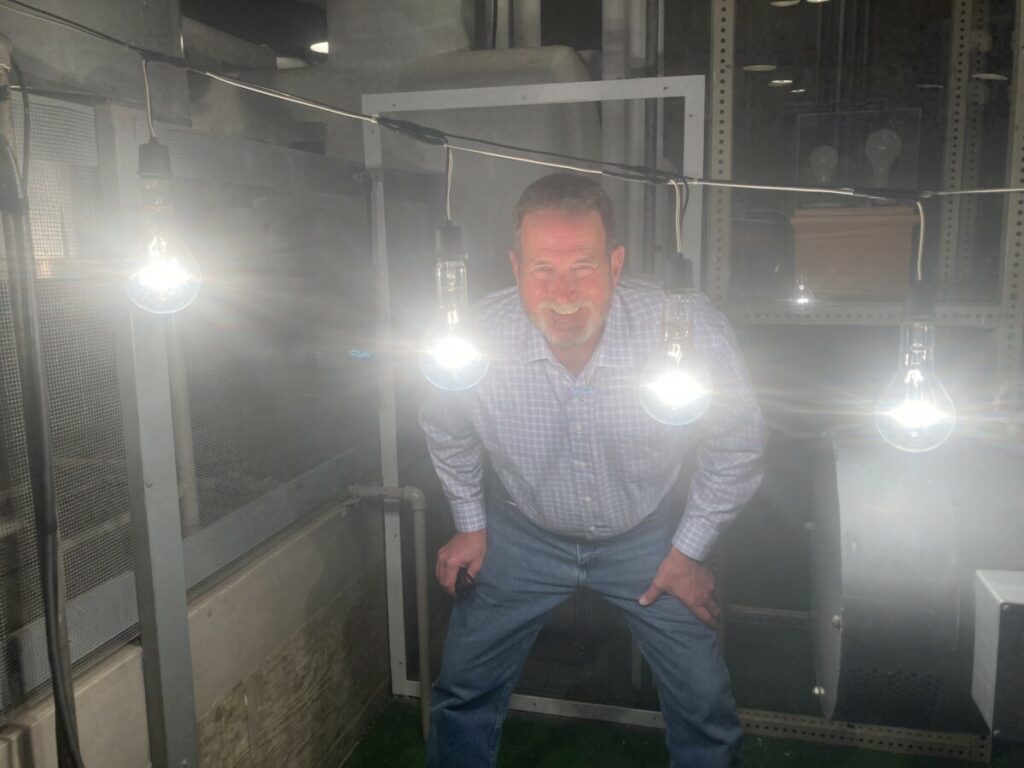
But since the dawn of the First Atomic Age, the primary design trend has been to strive for ever larger units in hopes of reducing the cost of the electricity they generate. In the 1950s, 60s and 70s, engineering degree programs taught that the “economy of scale” meant that equipment cost did not grow as rapidly as capacity.
For example, a pump that could produce ten times as much flow should only cost four times as much based on cost computations using input materials.
Design engineers learned by experience that bigger factories, bigger refineries and bigger mines could produce cheaper commodity products.
Seeing nuclear power plants as electricity factories, they could not help but believe that achieving economy of scale required them to design ever larger reactors and to place them in increasingly large groups at massive power stations.
There is a diseconomy of scale with super-sized units

Larger units can successfully use the economy of scale to lower the cost per unit of output but it isn’t the only kind of scale that can drive down costs. Ever larger units can also run into diseconomies of scale that plague mega-projects in construction, mass transit, sports complexes, and airports.
The experience of the industry in building the Vogtle AP1000s shows that there is such a thing as too large. In contrast, the economies of scale that we believe will aid in the appeal of SMRs takes the form of mass production and is expected to enable the construction of SMRs to more closely follow the declining cost curves experienced by wind and solar projects.
How can smaller reactors be produced and operated economically?
After observing the challenges associated with building only very large nuclear plants, there is a growing field of nuclear system developers who consider nuclear power plants to be a product, not a factory. A phrase that is repeatedly heard at gatherings of modern nuclear system developers is “We want to build airplanes, not airports.”
Entrepreneurial companies that see nuclear power systems as products understand that “scale” means building large quantities of the same product. They need to be positioned to meet the needs of a sufficient number of customers who want to buy enough machines to provide the opportunity to capture cost reductions from “experience curves” where cost declines as a result of cumulative product volume.
One advantage of smaller systems is the improved ability to use factory manufacturing techniques. Of course, the components used in conventional large reactors are produced in factories, but then they are individually shipped to the site to be assembled into an operating plant. With reactors that have the size and complexity closer to that of large ships or commercial aircraft, it is possible to assemble and transport complete or nearly complete products.
Factory workforces have many advantages over site construction workforces. They can improve productivity by repeating similar tasks regularly, They can live and work in cities served by mass transit. They can implement quality assurance techniques and environmental consistence systems that are difficult to achieve at remote large plant assembly sites.
Several of the entrepreneurial advanced nuclear companies are looking to scale their businesses using a hybrid approach. They recognize the need to build large quantities of their power- and heat-generating machines but they have found that there’s a limited universe of customers who want to operate nuclear power systems under current and foreseeable oversight regimes.
One solution to this problem is “Energy as a Service.” Companies can build, own and operate a fleet of their own small modular reactors to produce electricity and heat as the products they sell to willing customers. At least some of the companies following this path are keenly aware that there are numerous advantages to co-locating a significant number of the members of their nuclear fission fleets on each site they develop.
That statement is especially true under current regulatory requirements for security, oversight and quality assurance. Large numbers of smaller units on a single site also take advantage of repeatable, consistent work for the inevitable site-specific parts of erecting a power station. They can share transmission and cooling infrastructure, training, emergency response and administrative facilities.
One more variation on the SMR business model is the detectable emergence of developers that want to build on skills they’ve gained in deploying, owning and operating other kinds of power systems. They believe that certain SMRs, once designs are approved, can be readily integrated by project developers that specialize in engineering, permitting, construction, local politics, working with regional operators, and developing logistical supply chains for projects that blend clean energy production for local grids with water purification, carbon capture, hydrogen production and other revenue opportunities.
What about the waste for small modular reactors?
Lindsay Krall, Rodney Ewing and Allison Macfarlane recently published a paper titled Nuclear Waste from Small Reactors in the Proceedings of the National Academies of Science describing the larger surface to volume ratio of smaller reactors as a disadvantage.
Aside: Neutron Bytes published an article with extensive commentary on the study; though there is some overlap between that article and this one, this contains a few additional points of interest. End Aside.
The paper authors chose to conduct their study using publicly available information on three (out of dozens) small modular reactor designs. They looked at an early version of the NuScale Power Module, Terrestrial Energy’s IMSR™, and Toshiba’s 4S (a reactor system that has not been actively marketed since 2011.)
To the surprise of the lead author, that paper received unusual attention from the press.
I didn’t really know how the article would be released. There was a copy of the paper circulated to the media or to the press some five days in advance of the article’s publication. So, reactor developers were contacted by the press about the article before it was even published. As a scientist, I was just thinking, “Oh, thank God, this paper got accepted, and I don’t have to work with it anymore.” But then the release of the paper shocked me.
Diaz-Maurin,François Interview: Small modular reactors get a reality check about their waste, Bulletin of the Atomic Scientists, Jun 17, 2022
The attention was likely related to press releases issued by the host institutions before the study was actually published.
Both NuScale and Terrestrial Energy challenged the study’s conclusions about their systems. Dr. Jose Reyes, NuScale CTO, wondered about the way advanced copies of the study were released to the press and asked PNAS editor-in-chief May R. Berenbaum if PNAS had implemented a new policy about paper promotion.
It seems likely that summaries of the paper’s tenuous conclusions will be repeatedly introduced into discussions of small modular reactors. If not fully understood, the assertions about increased waste production might hamper SMR deployment.
The paper stated that smaller cores leak a larger portion of the fission neutrons and those neutrons activate structural materials. It also stated that increased neutron leakage leads to lower fuel efficiency because a reactor with increased neutron leakage needs a higher concentration of fissile material to maintain criticality.
Discussions with several nuclear design engineers that are not working on SMRs confirmed that those statements contained some truth and were worth consideration, but also described how they did not tell the complete story about waste generation from smaller nuclear plants.
Those design engineers pointed out that a marginal increase in the amount of waste generated per unit energy output would not make much of a difference in the effort needed to address long term storage or disposal of radioactive materials.
The paper expressly ignored the potential to reduce radioactive waste by recycling material and fuel.
This study also neglects to consider reprocessing, recycling, and dilution because these treatments will not eliminate the need for the storage, transportation, treatment, and disposal of radioactive materials.
Krall, L.M.; Macfarlane A.M.; Ewing, R. C. “Nuclear waste from small modular reactors” PNAS Vol. 119, No. 23 May 31, 2022
In her interview with the Bulletin of the Atomic Scientists, Dr. Krall pushed back on the headlines that emphasized the high end of the range of estimates offered. She said that high end estimate of increased waste generation only applied to sodium, a coolant proposed for use by several small and advanced reactor developers.
…for a sodium-cooled reactor, for instance, that sodium coolant is likely to become low-level waste at the end of the reactor’s lifetime, because it becomes contaminated and activated during reactor operation. So, the “up to 30 times more waste” that’s been driving the headlines, it’s mostly the sodium coolant.
Diaz-Maurin,François Interview: Small modular reactors get a reality check about their waste, Bulletin of the Atomic Scientists, Jun 17, 2022
For sodium waste, reuse in new reactors has the potential for a dramatic decrease in waste that needs to be processed for disposal, but only if there is a growing population of sodium-cooled reactors.
The potential for reuse of sodium from reactors is limited. The most likely utilization would be as a reactor coolant, but there are currently no LMFRs being constructed where sodium waste is available. (Emphasis added)
IAEA Radioactive Sodium Waste Treatment and Conditioning, Jan 2007. p. 46
Small reactors also have some advantages in fuel and nuclear plant material systems that include recycling. They are going to be easier to disassemble for some of the same reasons that they will be easier to assemble. Recycling factories can be less costly when designed to handle a more steady flow of smaller pieces and parts.
The Krall et. al. study also did not credit advanced reactors with fuel efficiency gains that arise from using operating temperatures that are significantly higher than those that are possible in large light water reactors.
Few of the teams designing SMRs point to reductions in waste generation as a major selling point, but quite a few of the designers point out the potential that fast reactors have for using recycled materials from the current used nuclear fuel stockpile and describe the efficiency gains from reactor systems that are capable of achieving much higher temperatures than conventional light water reactors.
Engineering is a profession that recognizes tradeoffs. Improvements in one design measure often results in a reduced performance in another design measure. Establishing priorities and determining the best overall choices requires expert, detailed knowledge that often isn’t available to outside academics. Small modular reactor developers and their backers have evidently determined that improving passive heat removal, constructibility, financial performance and customer acceptance are more important than the potential for a marginal increase in waste generation.
Waste from smaller modular reactors needs to be well managed, but neither the waste nor the misleading media headlines that appear to detract from the clear benefits of this emerging technology, should be seen as significant hurdles to their successful development.